
혼합 비티 독소단백질과 아이코사노이드 생합성 억제자를 이용한 약효 증진 기술
초록
Bacillus thuringiensis (비티)의 약효를 증가시키기 위한 일환으로 Cry 독소단백질의 혼합효과를 검정하였다. 서로 다른 네 가지 비티 균주에서 분리된 Cry 독소단백질 추출물들은 각각 좁은 적용해충범위를 나타냈다. 이들 Cry 독소단백질을 혼합한 결과 적용범위가 현격하게 증가했다. Xenorhabdus nematophila (Xn) 세균 배양액은 조사된 모든 곤충의 세포성 면역을 억제하고 Cry 독소단백질의 살충력을 증가시켰다. 이 Xn 세균배양액을 혼합 Cry 독소단백질에 추가한 결과 적용해충범위와 살충력을 모두 증가시켰다.
Abstract
To enhance Bacillus thuringiensis (Bt) efficacy, four Cry toxins were purified from four different Bt strains and assessed in their combined efficacy. The Cry mixtures significantly expanded their target insect spectra. Bacterial culture broth of Xenorhabdus nematophila (Xn) significantly suppressed insect cellular immune response and increased Cry toxicity. The addition of Xn culture broth to Cry mixture significantly enhanced Bt efficacy in target insect spectrum and insecticidal activity.
Keywords:
Cry toxin, immunosuppression, Bacillus thuringiensis, Xenorhabdus nematophila키워드:
독소단백질, 면역억제, 비티, 곤충병원세균서 론
여러 환경에 서식하는 곤충은 다양한 병원 미생물의 공격에 노출된다. 이들을 방어하기 위해 곤충은 다양한 생리작용을 가지고 있지만, 이 가운데 가장 효율적 방어기작이 면역반응이다(Beckage, 2008). 곤충의 면역반응은 척추동물과는 달리 선천성 반응만 지니고 있다. 이 선천성 면역반응은 세포성 면역반응과 체액성 면역반응으로 구분된다. 세포성 면역반응은 식균작용, 소낭형성 및 피낭형성으로 주로 혈구세포에 의해 수행된다(Gillespie et al., 1997). 체액성 면역반응은 산화효소인 페놀옥시데이즈나 항생단백질에 의해 수행되는 방어용 화합물 반응이다(Park and Lee, 2012). 그러나 면역반응 자체가 많은 에너지를 소모하고 또 자체적으로 해로운 자기면역으로 나타날 수 있기에 엄격한 조절기작 하에 있다(Adamo, 2008).
비티(Bacillus thuringiensis) 세균은 그람양성균으로 포자를 형성할 수 있으며 이때 결정형(Crystal: Cry) 독소단백질을 생성한다(Roh et al., 2007). Cry 단백질은 플라스미드에 암호되어 있고, 이들의 종류에 따라 비티의 적용해충범위가 결정된다(Bravo et al., 2012). 약 700 종류의 Cry 단백질 유전자가 밝혀졌고(Crickmore et al., 2014), 이들의 추정 아미노산 서열의 40% 유사도 기준에 따라 적어도 70 종류의 서로 다른 Cry 부류(Cry1, Cry2 등)로 나뉘고, 다시 70% 유사도 기준으로 세부류(Cry1A, Cry1B 등)로 나뉘고, 다시 70-95%의 유사도 기준에 따라 세세부류(Cry1Aa, Cry1Ab 등)로 나뉘게 된다(Crickmore et al., 1998). Cry 독소단백질은 크게 두 양상으로 약 130 kDa 크기 대형 단백질과 65-70 kDa 크기의 소형 단백질로 구성되는 원시 독소단백질로 형성되며, 곤충 중장 단백질 분해효소에 의해 N 말단(소형의 경우) 또는 N과 C 말단(대형의 경우)의 가수분해 반응으로 약 60 kDa 크기의 생리활성을 지닌 활성 독소단백질로 가공된다(de Magd et al., 2001).
활성화된 독소단백질의 살충기작은 중장막의 수용체에 결합으로부터 시작된다. Cry1Ab의 경우 캐드헤린과 같은 높은 결합력(Kd=1 nM)을 갖는 세포막 단백질에 결합된 Cry는 이 단백질 domain I에 위치한 첫 번째 α 구조 부위가 제거되면서 Cry 올리고 형성이 일어나게 된다. 이후 이들 올리고 독소단백질 복합체는 결합력(Kd)이 약 0.6 nM 정도의 alkaline phosphatase 또는 aminopeptidase N에 결합하면서 중장막에 구멍을 뚫게 되고 이에 따라 세포 안팎의 삼투압충격으로 중장세포의 치사를 유도하게 된다(Bravo et al., 2011). 또 다른 과정으로 캐드헤린에 결합된 Cry 독소단백질은 이 수용체를 통한 세포내 신호전달과정으로 세포치사를 일으키게 할 수 있다(Zhang et al., 2006). 중장세포치사에 따라 비티 세균이 혈강으로 침입하여 패혈증을 유발하여 궁극적으로 곤충을 치사하게 할 수 있으나 독소단백질만으로도 곤충을 치사시킬 수 있다는 증거는 비티가 패혈증의 원인이라고만 단정 지을 수 없다(Bravo et al., 2005). 이 보다는 중장에 서식하는 여러 다양한 미생물들이 비티 독소단백질에 의해 와해된 중장세포벽을 뚫고 혈강으로 침입하여 패혈증을 일으켰을 것으로 해석된다(Broderick et al., 2006). 이때 곤충 혈림프의 면역작용은 이들 미생물 침입의 방어기능을 담당할 수 있다(Grizanova et al., 2014). 따라서 이 대상 곤충의 면역작용 억제는 비티의 살충력을 증가시킬 수 있는 연구전략으로 이용되었다(Rahman et al., 2004; Kwon and Kim, 2007; Broderick et al., 2010).
비교적 인축에 안전한 해충방제 인자로 여겨지는 비티 살충제가 형질전환작물체를 제외하고 살포용 약제 면에서 농민들에게 보다 널리 이용되지 않은 이유는 좁은 적용해충범위와 화학농약에 비해 낮은 살충력으로 제기된다(Eom et al., 2014). 본 연구는 이 두 한계를 극복하기 위해 Cry 독소단백질의 혼합과 벡큘로바이러스 또는 면역억제물질과 혼용의 서로 다른 전략들을 세웠다. Cry 독소단백질의 살충력을 증가시키려는 노력은 또 다른 비티의 살충독소성분인 vegetative insecticidal protein (VIP)을 혼합하여 시도되었다. Cry 독소단백질의 활성부위(N 말단 부위)와 VIP 단백질을 혼합하여 제조한 융합단백질은 각 독소단백질의 단독 살충력 또는 두 독소단백질의 혼합 살충력에 비해 높은 결과를 나타냈다(Dong et al., 2012). 따라서 Cry 독소단백질의 혼합은 서로 다른 중장 수용체 결합을 통해 살충력에 보완 또는 상승작용을 꾀할 수 있을 것으로 첫째 연구 가설을 세웠다. 둘째로는 비교적 작용점이 다른 벡큘로바이러스와의 혼합은 비티의 적용해충범위 및 살충력의 증가를 꾀할 수 있다는 연구 가설을 세웠다. 끝으로 면역억제물질의 첨가는 궁극적 비티의 치사작용인 패혈증 유발에 걸림돌인 대상곤충의 면역적용을 억제하는 효과로 살충력을 높일 수 있다는 셋째 연구 가설을 세웠다.
재료 및 방법
공시곤충
본 연구에서는 네 종류의 해충이 사용되었다. 파밤나방(Spodoptera exigua)은 1994년 파(Allium fistulosum) 재배지에서 채집하였고, 실내에서 인공사료(Goh et al., 1991)로 누대 사육을 하였다. 성충은 10% 설탕물을 먹이로 공급하였다. 배추좀나방(Plutella xylostella)은 안동시 송천동에 소재한 배추밭에서 유충을 채집하여, 약제 처리하지 않고, 실내에서 누대 사육한 것을 실내 약제 노출 실험에 사용하였다. 유충은 배추를 먹이로 주었으며 성충은 10% 설탕물을 먹이로 하였으며 배추를 갈아서 만든 배춧물로 산란을 유도하였다. 거짓쌀도둑거저리(Tribolium castaneum)는 밀가루, yeast extract (Scharlau, Barcelona, Spain), trypton (BD, NJ, USA) (100:50:50, g/g)을 먹이로 사육하였으며, 최종영기(7령)의 유충(체장 6-7 mm)을 분석에 이용하였다. 노랑초파리(Drosophila melanogaster)는 인스턴트 초파리배지(한솔테크, 서울, 한국)를 이용하여 사육을 하였다. 모든 곤충 사육은 온도 25 ± 1°C, 광주기 16:8 h (L:D), 상대습도 40~60%의 조건이었다.
곤충병원세균 균주 배양
곤충병원세균(Xenorhabdus nematophila: Xn)은 기주 선충에서 분리된 후 동결보관중인 것을 이용하였다(Jung and Kim, 2006). 이들 균주는 tryptic soy broth (TSB, MBcell, Seoul, Korea) 배지를 이용하여 28°C에서 12시간 동안 배양하여 단일 균총을 채취하였다. 채취된 단일 균총을 TSB 액체배지를 이용하여 28°C에서 16시간 동안 배양한 후, 글리세롤이 30%가 되도록 첨가하여 보관용 세균 균주를 만들었다. 이 보관 균주는 반복되는 세균 배양의 원시료로 이용되어 계대배양에 따른 세균 변이 가능성을 줄였다.
비티 균주는 네 종류의 비티를 이용하였다. B. thuringiensis subsp. kurstaki (BtK)는 슈리사이드® (바이엘 크롭사이언스 주식회사, 서울, 한국) B. thuringiensis subsp. aizawai (BtA)는 센타리® (전진산업 주식회사, 대전, 한국), B. thuringiensis subsp. israelensis (BtI)는 박토섹® (성인제약 주식회사, 서울, 한국), B. thuringiensis subsp. tenebrionis (BtT)는 미국 조지아대학교 Mike Adang 교수 연구실에서 분양 받았다. 각각의 균주에 멸균수 1 mL를 혼합하여 현탁액을 만든 후 루프를 이용하여 TSB 평면 배지에 세균을 도말하고 30°C에서 24시간 배양하였다. 평판배지에서 배양된 세균에서 단일 콜로니를 얻고 이를 멸균수로 희석하여 TSB 액체배지를 이용하여 28°C에서 48시간 동안 200 rpm에서 교반배양 후, 다시 48시간 동안 4°C 저온고에 보관하여 세균의 포자 형성율을 증가시켰다. 포자 형성은 위상차 광학현미경(BX-PHD, Olympus, Tokyo, Japan)을 이용하여 1,000배 배율에서 확인하였다.
Cry 독소단백질 분리
분리된 Bt 균주의 단일 균총을 2 mL의 Luria-Bertani(LB) medium에 접종한 후 28°C의 배양기에서 200 rpm으로 48시간 동안 교반 배양하였다. 이후 2 mL의 세균배양액을 1 L의 peptone glucose salt medium (Brownbridge and Margalit, 1986)에 접종하여 동일한 조건으로 72시간 동안 포자형성과 세포분해가 진행될 때까지 배양하였다. 배양 후 4,000 rpm에서 포자와 Cry 독소단백질 층을 수거한 후 0.2%의 lysozyme (Sigma, MO, USA)이 포함된 10 mL의 lysis buffer (0.1 M NaCl, 2% Triton X-100, 20 mM Bis-Tris, pH 6.5)에 현탁한 후 28°C의 배양기에서 200 rpm으로 1시간 동안 세포용해 반응을 진행하였다. 이후 2분간 초음파 파쇄기(Bendelin Sonoplus HD2070, Berlin, Germany)를 이용하여 95%의 출력으로 초음파 처리를 하여 균체로부터 Cry 독소단백질의 분리를 용이하게 하였다. 분리된 Cry 독소단백질과 포자는 4°C에서 10분간 12,000 rpm으로 원심분리한 후 상등액을 제거하고 멸균 3차증류수로 세척한 후 5 mL의 멸균 3차증류수에서 균일하게 현탁시켰다. 독소단백질 정량분석은 bovine serum albumin (Sigma, MO, USA)을 표준단백질로 10% SDS-PAGE에서 검정선을 얻은 후 비교 정량화하였다.
Xn 세균 배양물질 추출
Xn 세균을 TSB 배지를 이용하여 48시간 배양하였다. 분획깔때기에 1 L의 세균 배양액을 넣고 330 mL의 헥산을 넣고 마개로 막은 다음 30회 흔들어 주었다. 이러한 진탕 반응 동안 매 5회 진탕 시 코르크를 열어 가스를 배출했다. 이후 거치대에 separate funnel을 2시간 동안 방치한 후 헥산 추출 상층액을 회수하고, 다시 330 mL의 헥산을 첨가하고 동일한 방법으로 추출했다. 다시 340 mL의 헥산을 첨가하고 동일한 방법으로 추출했다. 수집된 헥산층은 무수황산나트륨을 500 g 넣은 후 탈수시켰다. 이후 2 L의 삼각플라스크 입구에 여과지를 설치하고 탈수시킨 유기용매 추출물들을 부어 무수황산나트륨을 제거시켰다. 이 추출물을 감압농축기를 이용하여 감압농축(40°C, 1시간)하여 용매층을 제거했다. 유기용매가 사라진 플라스크 벽면에 용출된 대사물질을 5 mL의 메탄올을 첨가하여 용해시켜 회수하였다.
재조합 벡큘로바이러스
Cry1Ac와 Cry1Ca 독소단백질이 재조합된 Autographa californica multiple nucleopolyhedrosis virus (AcMNPV)를 서울대학교 제연호 교수 연구실에서 분양받았다. Cry1Ca와 Cry1Ac에 특이적 유전자 프라이머(Cry1Ca: 5'-GCC AGT TGG TCA ACT AAG GGA AG-3'와 5'-CCG TAC CTC TTC CTC GAT ACG TAA A-3', Cry1Ac: 5'-GAT ATC GTT GCT CTG TTC CCG AA-3'와 5'-CAC GTT GTT ATT CTG TGG CGG T-3')를 이용해 98°C에서 30초, 55°C에서 30초, 72°C에서 30초간 PCR 반응을 30회 진행한 후 증폭된 밴드를 통하여 Cry 독소단백질의 차이를 확인하였다.
Cry 독소단백질 생물검정
생물검정은 Cry 독소단백질의 섭식을 통해 이루어졌다. 배추좀나방과 파밤나방을 검정할 경우 대상 곤충 개체 당 0.4 μg의 Cry 독소단백질을 배추 잎(1 × 1 cm)에 처리하였다. 노랑초파리는 사과껍질(0.5 × 0.5 cm)에 처리하였다. 거짓쌀도둑거저리는 먹이에 처리를 하였다. 처리하기 전 4시간 동안 시험곤충들을 절식시키고, 이후 처리 먹이를 모두 먹는 것을 확인한 후 무처리 먹이를 공급하였다. 처리구당 10마리씩 3반복으로 실시하였으며, 생존 확인은 24시간 주기로 7일차 까지 매일 하루 중 같은 시간에 조사하였다.
벡큘로바이러스 생물검정
벡큘로바이러스의 생물검정으류 위해 budded 형태의 바이러스를 0-106 pfu 농도로 혈강에 주입하였다. 경구 처리는 배추잎에 일정한 농도의 벡큘로바이러스를 처리하고 대상 유충에게 섭식시켜 살충율을 분석하였다. Sf9 세포주에서 증식된 다각체형 바이러스(7 × 107 PIB/ml) 10 μl를 5 ×5mm의 배추 잎 조각에 처리하였다. 이를 직경 3 cm의 원형 용기에 여과지를 깔고 올려놓았다. 처리된 배추잎 주변으로 10마리의 3령 유충을 옮겨 놓고 처리된 잎이 모두 섭식되도록 5시간 방치하였다. 동일한 처리가 3반복되었다. 이후 3-4마리씩 나누어 배추좀나방은 배추, 파밤나방은 인공사료로 사육되었다. 처리 후 24시간 마다 치사된 개체를 조사하였다. 이때 치사 유충은 외부 자극에 대해서 능동적 반응 움직임이 없는 개체로 규정하였다.
혈구 소낭형성분석
세포성 면역반응의 일환으로 세균 침입에 따른 혈구의 소낭형성 반응을 네 종류의 곤충에 대해서 분석했다. 대장균(E. coli Top10)을 LB 배지에서 증식시키고 5 × 104 세균 세포수를 대상 곤충의 혈강으로 주입하였다. 혈강 주입은 유리 모세관을 이용하여 초미량펌프가 장착된 미세조정장치(SYS-microcontroller, World Precision Instruments, Sarasota, FL, USA)를 이용하여 주입하였다. 유리 모세관은 micropipette puller (PN-30, Narishige, Tokyo, Japan)를 이용하여 제조하였다. 처리 Xn 추출물은 세균 주입하기 1시간 전에 소정의 농도로 주입했다. 세균이 주입된 유충은 곤충 증식 조건(온도 25 ± 1°C, 광주기 16:8 h (L:D), 상대습도 40~60%)에서 8시간 배양시켰다. 이후 4°C로 옮긴 후 소낭형성 반응을 정지시켰다. 이후 각 처리 곤충을 해부하여 형성된 소낭을 해부현미경 50배 배율에서 관찰했다. 형성된 소낭수는 먼저 소화관 주변 및 기관지에 붙어 있는 것들을 계수하고, 이후 소화관을 적출하고 가려진 몸 부위에 존재했던 소낭을 추가로 계수하여 산출하였다. 각 소낭 분석은 처리 개체를 반복으로 5마리에 각 처리가 실시되었다.
통계처리
모든 살충효과 시험 결과는 백분율 자료로서 arsine 변환 후 SAS의 PROC GLM (SAS Institute, 1989)을 이용하여 ANOVA 분석 및 처리 평균간 비교를 실시하였다.
결 과
비티 Cry 독소단백질 분리와 살충효과 특이성
Cry 독소단백질이 네 종류의 비티 균주로부터 각각 분리되었다(Fig. 1A).이들 분리단백질들을 SDS-PAGE를 이용하여 분석한 결과 BtK, BtA, BtI는 약 130 kDa 그리고 BtT는 약 70 kDa에서 주요 단백질 밴드를 나타냈다. 그러나 모든 분리 시료에서 주 단백질 밴드 외에 다른 단백질이 미량으로 존재했다. 이들 단백질의 본체는 본 연구에서 밝혀지지 않았다.
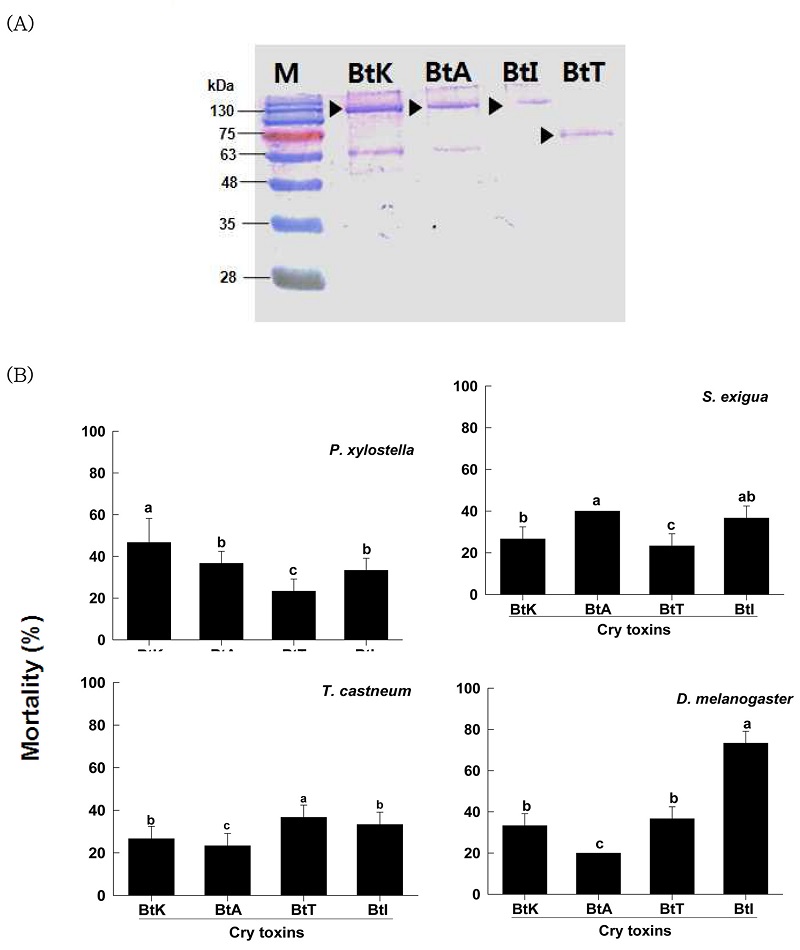
Specific toxicity of different Cry toxins. (A) Purification of Cry toxins from four different Bacillus thuringiensis: B. thuringiensis kurstaki (‘BtK’), B. thuringiensis aizawai ('BtA'), B. thuringiensis tenebrionis (‘BtT’), and B. thuringiensis israelensis (‘BtI’). The purified Cry toxins were separated on 10% SDS-PAGE. Each lane contained 10 衫 of Cry toxin proteins. Arrow heads indicate main Cry toxin proteins. (B) Insecticidal activities of different Cry toxins against lepidopteran, coleopteran, and dipteran insects. Third instar larvae of Plutella xylostella, Spodoptera exigua, and Drosophila melanogaster were used for bioassay, while seven instar larvae of Tribolium castaneum were used. Each larva was fed in a dose of 0.4 衫 Cry toxin. Each replication consisted of 10 larvae. Each treatment was replicated three times. Mortality was assessed for 7 days after treatment at 25°C. Different letters above standard deviation bars indicate significant difference among means at Type I error = 0.05 (LSD test).
분리된 Cry 독소단백질들의 서로 다른 해충에 대한 살충력을 비교하였다(Fig. 1B). 조사 대상인 네 종류의 곤충에 대해 이들 네 종류의 Cry 독소단백질은 뚜렷한 살충력을 나타냈다. 그러나 각 Cry 독소단백질은 서로 다른 곤충에 대해서 상이한 살충력을 나타냈다. BtK는 배추좀나방에 대해서 다른 곤충에 비해 높은 살충력을 나타냈다. 유사하게 BtA는 파밤나방, BtT는 거짓쌀도둑거저리 그리고 BtI는 노랑초파리에 대해서 각각 상대적으로 높은 살충력을 나타냈다. 그러나 노랑초파리에 대한 BtI의 살충력을 제외하고 모두는 50%에 미치지 않은 낮은 살충력을 보였다.
Cry 독소단백질 혼합과 적용해충범위 변화
서로 다른 Cry 독소단백질을 혼합하여 적용해충범위 변화를 분석했다(Fig. 2). 두 종류의 Cry 독소단백질을 혼합한 결과 혼합된 Cry 독소단백질 종류에 따라 적용해충범위가 결정되는 것을 나타냈다(Fig. 2A). 예를 들어, BtK와 BtA 유래 Cry 독소단백질을 혼합하면, 파밤나방과 배추좀나방에 대해서 다른 두 해충에 비해 높은 방제 효과를 가져 주었다. 세 종류의 Cry 독소단백질을 혼합한 결과도 포함된 Cry 독소단백질의 종류에 따라 적용해충의 범위가 변동되었다(Fig. 2B). 예를 들어, BtK, BtA, BtK 유래 Cry 독소단백질을 혼합한 결과 파리류에 유효성분인 BtI의 독소단백질이 누락되어 노랑초파리에 대한 살충력은 낮은 것으로 나타났다. 따라서 4 종류의 Cry 독소 단백질을 모두 혼합한 결과 통계적으로 차이가 있지만, 비교적 4 가지 해충에 대해 높은 (> 50%) 살충력을 나타냈다.
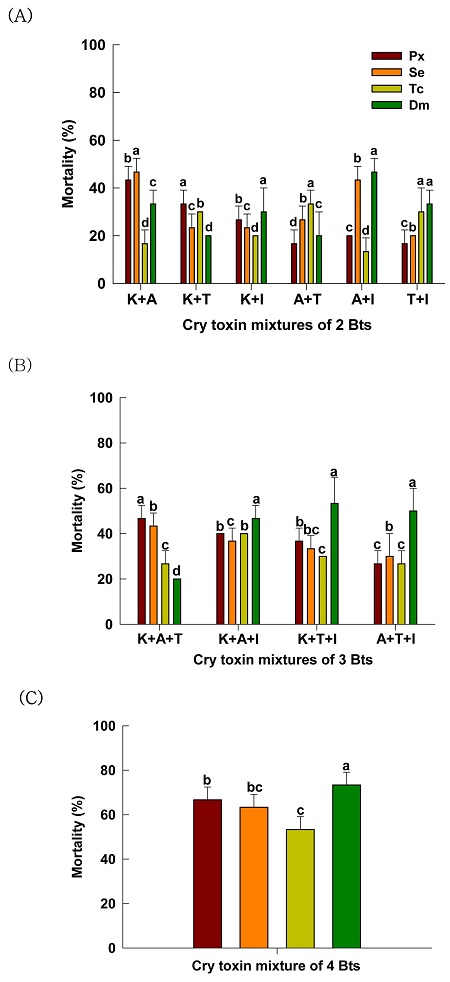
Effect of Cry toxin mixture on target insect spectrum. (A) Two Cry toxin mixture (B) Three Cry toxin mixture (C) Four Cry toxin mixture. Cry toxins from four different Bacillus thuringiensis (Bt): B. thuringiensis kurstaki (‘K’), B. thuringiensis aizawai (‘A’), B. thuringiensis tenebrionis (‘T’), and B. thuringiensis israelensis (‘I’). Third instar larvae of Plutella xylostella (‘Px’), Spodoptera exigua (‘Se’), and Drosophila melanogaster (‘Dm’) were used for bioassay, while seven instar larvae of Tribolium castaneum (‘Tc’) were used. Each larva was fed in a dose of 0.4 μg Cry toxin mixture. Each replication consisted of 10 larvae. Each treatment was replicated three times. Mortality was assessed for 7 days after treatment at 25°C. Different letters above standard deviation bars indicate significant difference among means at Type I error = 0.05 (LSD test).
벡큘로바이러스를 이용한 Cry 독소단백질 적용해충범위 변화
Cry 독소단백질의 적용해충범위를 넓히는 데 벡큘로바이러스의 효과를 분석하고자, Cry1Ac와 Cry1Ca 독소단백질 유전자를 각각 재조합한 AcMNPV 바이러스를 이용하였다(Fig. 3). 대조구로서 형광단백질(‘EGFP’)을 발현하는 재조합 AcMNPV를 사용하였다. 우선 재조합벡큘로바이러스에 Cry1Ac와 Cry1Ca 유전자의 존재를 독소단백질 특이적 프라이머를 이용하여 PCR로 확인하였다(Fig. 3A). 바이러스 단독에 비해 Cry 독소단백질을 발현하는 재조합 벡큘로바이러스가 두 해충 모두에서 높은 살충력을 나타냈다(Fig. 3B). 그러나 Cry 독소단백질을 발현하는 재조합벡큘로바이러스에서는 Cry 독소단백질에 따라 대상 해충에 미치는 살충력이 상이했다. 즉, BtK에서 유래된 Cry 독소단백질의 주성분인 Cry1Ac와 재조합된 AcMNPV는 배추좀나방에 대해서 높은 살충력을 보인 반면 BtA의 주요 Cry 독소단백질인 Cry1Ca를 발현하는 AcMNPV는 파밤나방에 대해서 높은 살충력을 나타냈다.
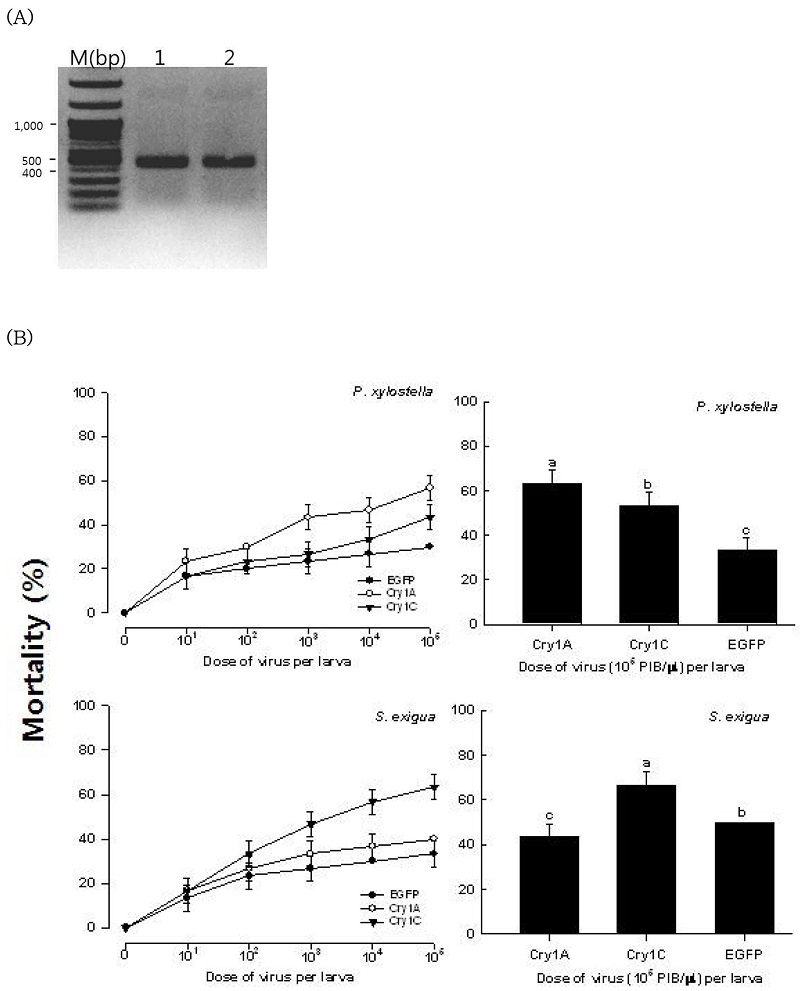
Effect of baculovirus expressing Cry toxins. (A) Confirmation of baculoviruses expression Cry1Ca (lane 1) and Cry1Ac (lane 2). (B) Insecticial effects of Cry-expression bacluviruses against two different lepidopteran insects of Plutella xylostella and Spodoptera exigua by injection (left panels) or feeding (right panels) bioassay. For injection assay, each larva was fed in different doses (pfu) of virus. For feeding assays, each larva was injected with 106 PIB of virus. Each replication consisted of 10 larvae. Each treatment was replicated three times. Mortality was assessed for 7 days after treatment at 25°C.
면역억제물질과 Cry 독소단백질의 독성 변화
Xn 세균 배양액 추출물은 곤충면역억제물질을 포함하고 있다(Seo et al., 2012). 이 추출물이 다양한 곤충의 면역억제를 유도할 수 있는 지 세포성 면역반응인 혈구의 소낭형성반응으로 분석하였다(Fig. 4A). 배추좀나방의 경우는 비병원성세균인 대장균 주입에 따라 약 42개의 소낭을 형성했다. 그러나 Xn 추출물을 함께 주입할 경우 5개 미만의 소낭을 발현하여 약 88%의 면역억제효과를 나타냈다. 유사하게 Xn 추출물은 파밤나방에게 약 91%, 거짓쌀도둑거저리에게 약 68% 그리고 노랑초파리에게 약 92%의 면역억제효과를 나타냈다.
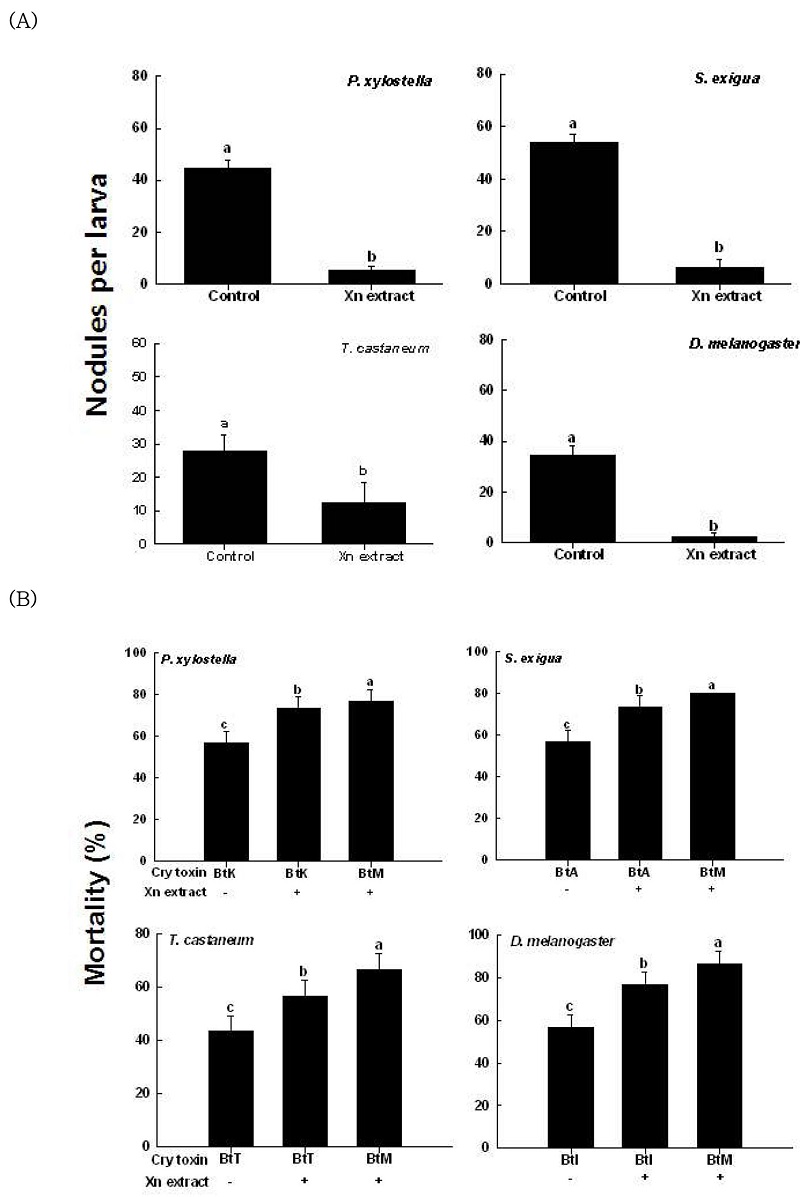
Immunosuppression and enhancement of Cry toxin toxicity. (A) Nodulation assay to assess the immunosuppressive activity of Xenorhabdus nematophila (‘Xn’) culture broth extract. Xn extract was prepared by organic solvent extracts. Each treatment was replicated five times. (B) Enhancement of Cry toxicity by addition of Xn extract. Cry toxins from four different Bacillus thuringiensis: B. thuringiensis kurstaki (‘BtK’), B. thuringiensis aizawai (‘BtA’), B. thuringiensis tenebrionis (‘BtT’), and B. thuringiensis israelensis (‘BtI’). 'BtM' indicates a mixture of four different species. Third instar larvae of Plutella xylostella, Spodoptera exigua, and Drosophila melanogaster were used for bioassay, while seven instar larvae of Tribolium castaneum were used. Each larva was fed in a dose of 0.4 μg Cry toxin. Each replication consisted of 10 larvae. Each treatment was replicated three times. Mortality was assessed for 7 days after treatment at 25°C. Different letters above standard deviation bars indicate significant difference among means at Type I error = 0.05 (LSD test).
이 Xn 추출물을 Cry 독소단백질과 혼합하여 살충효과 증대 및 적용범위 확대를 분석하였다(Fig. 4B). BtK 유래 Cry 독소단백질에 Xn 추출물을 혼합한 결과 살충력은 약 75%까지 증가하였다. 네 가지 Cry 독소단백질의 혼합체(‘BtM’)에 Xn 추출물을 혼합한 결과 약 79%까지 방제 효과가 증가했다. 파밤나방의 경우에도 Xn 추출물은 BtA 유래 Cry 독소단백질은 물론이고 Cry 독소단백질 혼합체에 방제 효과를 현격하게 증가시켰다. 유사한 결과가 거짓쌀도둑거저리와 노랑초파리에서 나타났다. 즉, Cry 독소단백질 복합체가 Xn 추출물과 혼합된 경우 조사된 모든 해충에 대해서 높은 살충력을 나타냈다.
고 찰
저항성관리의 기본 원리로서 해충의 저항성 발달 속도를 늦추기 위해서는 비티를 고독성의 복합 독소단백질 구성이 필요하다(Park et al., 2005). 이에 입각하여 기존 비티에 B. sphaericus 유래의 Bin 독소단백질과 BtA 유래의 Cyt1A 단백질을 복합시킨 결과 이들의 상승효과에 따라 높은 방제효과를 나타냈다. 또한 비티 감염에 따라 나타나는 곤충의 면역저항성을 억제하는 것이 비티의 효율을 높이는 데 유효하다(Kwon and Kim, 2007). 본 연구는 다양한 비티 균주에서 분리된 Cry 독소단백질을 분리하고, 이들의 혼합에 따라 살충력 증가 및 적용해충범위를 넓히려 시도되었다. 또한 벡큘로바이러스를 이용하여 Cry 독소단백질의 특이성을 줄이고 적용해충범위를 넓히려 시도하였다. 마지막으로 면역억제물질을 생성하여 분비하는 Xn 세균 배양액을 이용하여 비티의 살충효과 증가 및 적용범위 확대를 꾀하였다.
Cry 독소단백질의 복합체는 적용해충범위를 증가시켰다. 각 Cry 독소단백질은 대상 곤충에 대한 특이적 살충 효과를 나타냈다. 이러한 이유는 Cry 단백질의 작용점인 중장 수용체의 특이성에서 기인된 것으로 사료된다. 즉, Cry 독소단백질의 복합체는 다양한 비티 수용체에 결합할 수 있는 Cry 독소단백질을 제공한 것은 물론이고, 이들 Cry 독소단백질 사이에 상호 작용에 기인된 상승효과도 이러한 적용범위확대에 기인된 것으로 해석된다. 유사한 연구로서 Cry 독소단백질의 살충력을 증가시키려는 노력은 또 다른 비티의 살충 독소성분인 vegetative insecticidal protein (VIP)을 혼합하여 시도되었다. Cry 독소단백질의 활성부위(N 말단 부위)와 VIP 단백질을 혼합하여 제조한 융합단백질은 각 독소단백질의 단독 살충력 또는 두 독소단백질의 혼합 살충력에 비해 높은 결과를 나타냈다(Dong et al., 2012). 이는 융합 독소단백질의 Cry 독소단백질의 알칼리 조건에서 용해도를 높여 주어 나타난 결과로 해석되었다. 유사한 독소단백질의 혼합 상승효과는 B. sphaericus가 영양번식기에 생산하는 모기 독소단백질과 Cry 독소단백질의 혼합처리에서도 증명되었다(Wirth et al., 2007).
벡큘로바이러스의 혼합에 따른 Cry 독소단백질의 적용해 충범위 증가 효과는 본 연구에서는 관찰되지 않았다. 비록 두 생물제제의 혼합에 따라 각 대상 곤충에 대한 살충력은 증가되었으나 적용해충 증가는 나타나지 않았다. 일반적으로 벡큘로바이러스 병원성은 중장을 통한 침입 이후 중장에 붙어 있는 기관지세포로 전이되어 온몸으로 전파되는 것으로 알려지고 있다(Washburn et al., 1995, 2001; Kirkpatrick et al., 1998). 즉, 중장세포가 벡큘로바이러스 체내 침입의 주요 관문이 되어, 이들 세포의 항바이러스 기작은 이에 대한 병원성을 낮출 수 있다. 여기서 Cry 독소단백질의 작용으로 상호 상승작용을 기대했었지만, 비적용곤충의 경우 이 단백질의 특이적 수용체의 부족으로 벡큘로바이러스의 병원력을 증가시키지 못한 것으로 해석된다. 즉, 벡큘로바이러스의 작용 속도가 상대적으로 느리기 때문에 Cry 독소단백질의 특이성을 해결하여 주지 못했을 것으로 사료된다.
면역억제물질이 포함된 Xn 추출물은 Cry 독소단백질의 살충력을 크게 증가시켰다. Xn 세균은 그람음성균으로 선충의 장내에 서식하고 있으며, 기주 선충이 대상 곤충의 혈강으로 침입하면, 선충 장속에서 곤충의 혈강으로 빠져나와 다양한 곤충병원기작을 발휘하게 된다(Akhurst, 1980; Kaya and Gaugler, 1993). 특별히 Xn은 감염 초기에 대상 곤충의 세포성 및 체액성 면역을 모두 억제하게 된다(Park and Kim, 2003; Hwang et al., 2013). 이러한 Xn의 곤충 면역억제 기작은 주로 곤충의 면역 중개물질인 아이코사노이드 생합성을 억제하면서 나타나게 된다(Kim et al., 2005). 아이코사노이드는 탄소수 20개의 다가불포화지방산의 산화물들이다. 이들 물질은 주로 인지질을 기질로 인지질분해효소의 일종인 phospholipase A2 (PLA2)의 촉매작용으로 유리된 아라키도닉산(5,8,11,14-eicosatetraenoic acid)이 cyclooxygenase 또는 lipoxygenase의 작용으로 유도된 프로스타글란딘류 및 류코트리엔류가 곤충에서 나타난다(Stanley and Kim, 2014). 따라서 PLA2는 아이코사노이드 생합성 과정의 첫 반응을 촉매하는 효소로서 Xn의 억제 대상이 된다(Shrestha and Kim, 2009). Xn의 세균배양액에 PLA2 억제 물질이 포함되었으며, 이 물질은 유기용매로 추출되었다(Park et al., 2004a,b). 일련의 분획구 분리 방법을 이용하여 이 세균 배양액에 존재하는 PLA2 억제 물질을 분리하여 총 8개 화합물의 물질 구조를 동정하였다(Seo et al., 2012). 본 연구에서 Xn 추출물은 나방류는 물론이고 파리류 및 딱정벌레에까지 면역억제를 나타냈다. 이러한 면역억제효과에 따라 Cry 독소단백질의 살충력 증가 요인은 Cry 독소단백질의 중장 파괴 이후 일어나는 장내 세균의 혈강 침입을 대상 곤충이 효과적으로 방어하지 못해 비교적 용이하게 패혈증이 유발된 것으로 해석된다. 유사한 연구로서 지중해밀가루명나방(Ephestia kuehniella)에 대한 비티 살충력이 Xn과 혼합처리에 의해 증가되었다(BenFarhat et al., 2013). 특별히 Cry 독소단백질 종류에 따라 이러한 상승효과가 상이하였는데, 주된 이유는 Cry 독소단백질이 중장의 수용체에 대한 친화력이 높을수록 Xn의 상승효과는 높아졌다(BenFarhat et al., 2013). 즉, Cry 독소단백질에 의해 중장의 세포붕괴는 Xn으로 하여금 혈강으로 침입할 기회를 제공하여 이 두 세균의 상승효과를 설명한 Jung and Kim (2006)의 결과를 뒷받침하고 있다. 또한 면역억제를 통한 비티의 살충력 증가의 다른 예로서 Cry3Ba를 거짓쌀도둑거저리에 처리된 경우 apolipophorin III (ApoLpIII) 발현을 2.1 배 증가시켰으며, 이 유전자의 발현을 RNA interference로 억제시킨 경우 이 비티 독소단백질에 대한 감수성이 현격하게 증가되었다(Contreras et al., 2013). 한편, 파밤나방에 Xn을 처리한 경우 ApoLpIII의 발현을 억제시켰다(Hwang et al., 2013). 따라서 본 연구에서 Xn이 거짓쌀도둑거저리에 대해 BtT 유래 Cry 독소단백질의 살충력을 증가시킨 것은 Xn, 대사물질이 ApoLpIII 발현을 억제하여 면역저하를 유도하고 이에 따라 BtT 유래 Cry 독소단백질의 활성을 높인 것으로 해석된다. 본 연구에서 여러 Cry 독소단백질에 대한 Xn 대사물질의 상승효과는 Cry 독소단백질의 대상 곤충의 중장세포층 파괴와 이에 따라 Xn이 갖는 다양한 면역억제물질에 따라 궁극적 패혈증 효과의 증가로 해석된다.
비티의 약효증가 및 저항성 문제를 해결하기 위해 다른 생물농약 살충제와 혼합 처리가 여러 사례에서 찾아 볼 수 있다. 이 가운데 neem 오일의 주성분인 azadirachtin과 혼합효과가 왕담배나방(Helicoverpa armigera)에 대해서 분석되었고 뚜렷한 상승효과를 나타냈다(Singh et al., 2007). 또 다른 혼합처리 형태로서 비티 형질전환 작물과 내부기생봉과의 조합이 시도되었다(Vojtech et al., 2005). 특별히 비티는 물론이고 화학농약에 저항성인 배추좀나방에 대해서 프루텔고치벌(Cotesia plutellae)은 감수성 개체와 차이 없이 기생 선호성을 보여 이들 혼합 방제의 가능성을 높여 주었다(Kim et al., 2013). 본 연구는 이러한 생물방제제들의 혼합 처리에 의한 비티 살충력 증가는 물론이고, Cry 독소단백질의 혼합에 의한 적용해충범위 증가를 꾀할 수 있다는 실험적 자료를 제공하고 있다.
Acknowledgments
본 연구는 농림축산식품부 생명산업기술개발사업에 의해 이루어졌습니다.
Literature Cited
- Adamo, S. A., (2008), Bidirectional connections between the immune system and the nervous system in insects, In Insect immunology BeckageN. E.>EdsAcademic Press, New York, p129-149.
-
Akhurst, R. J., (1980), Morphological and functional dimorphism in Xenorhabdus spp., bacteria symbiotically associated with the insect pathogenic nematodes Neoaplectana and Heterorhabditis, J. Gen. Microbiol, 121, p303-309.
[https://doi.org/10.1099/00221287-121-2-303]
- Beckage, N. E., (2008), Insect immunology, p348, Academic Press, New York.
-
BenFarhat, D., M. Danmark, S. B. Khedher, S. Mahfoudh, S. Kammoun, and S. Tounsi, (2013), Response of larval Ephestia kueniella (Lepidoptera: Pyralida) to individual Bacillus thuringiensis kurstaki toxins mixed with Xenorhabdus nematophila, J. Invertebr. Pathol, 114, p71-75.
[https://doi.org/10.1016/j.jip.2013.05.009]
-
Bravo, A., S. S. Gill, and M. Soberón, (2005), Bacillus thuringiensismechanisms and use, In Comprehensive molecular insect science, L. I. Gilbert, K. Iatrou, and S. S. Gill Eds, Elsevier, New York, p175-206.
[https://doi.org/10.1016/B0-44-451924-6/00081-8]
-
Bravo, A., I. Gómez, H. Porta, B. I. Garcia-Gómez, C. Rodriguez-Almazan, L. Pardo, and M. Soberón, (2012), Evolution of Bacillus thuringiensis Cry toxins insecticidal activity, Microbial Biotechnol, 6, p17-26.
[https://doi.org/10.1111/j.1751-7915.2012.00342.x]
-
Bravo, A., S. Likitvivatanavong, S. S. Gill, and M. Soberón, (2011), Bacillus thuringiensis: a story of a successful bioinsecticide. Insect Biochem, Mol. Biol, 41, p423-431.
[https://doi.org/10.1016/j.ibmb.2011.02.006]
-
Broderick, N. A., K. F. Raffa, and J. Handelsman, (2006), Midgut bacteria required for Bacillus thuringiensis insecticidal activity, Proc. Natl. Acad. Sci. USA, 103, p15196-15199.
[https://doi.org/10.1073/pnas.0604865103]
-
Broderick, N. A., K. F. Raffa, and J. Handelsman, (2010), Chemical modulators of the innate immune response alter gypsi moth larval susceptibility to Bacillus thuringiensis, BMC Microbiol, 10, p129.
[https://doi.org/10.1186/1471-2180-10-129]
-
Brownbridge, M., and J. Margalit, (1986), New Bacillus thuringiensis strains isolated in Israel are highly toxic to mosquito larvae, J. Invertebr. Pathol, 48, p216-222.
[https://doi.org/10.1016/0022-2011(86)90126-6]
-
Contreras, E., C. Rausell, and M. D. Real, (2013), Tribolium castaneum apolipophorin-III acts as an immune response protein against Bacillus thuringiensis Cry3Ba toxic activity, J. Invertebr. Pathol, 113, p209-213.
[https://doi.org/10.1016/j.jip.2013.04.002]
- Crickmore, N., D. R. Zeigler, J. Feitelson, E. Schnepf, J. Van Rie, and D. Lereclus, (1998), Revision of the nomenclature for the Bacillus thuringiensis pesticidal crystal proteins, Microbiol. Mol. Biol. Rev, 62, p807-813.
- Crickmore, N., J. Baum, A. Bravo, D. Lereclus, K. Narva, K. Sampson, E. Schnepf, M. Sun, and D. R. Zeigler, (2014), 'Bacillus thuringiensis toxin nomenclature', http://www.btnomenclature.info.
-
de Máagd, R. A., A. Bravo, and N. Crickmore, (2001), How Bacillus thuringiensis has evolved specific toxins to colonize the insect world, Trends Genet, 17, p193-199.
[https://doi.org/10.1016/S0168-9525(01)02237-5]
-
Dong, F., R. Shi, S. Zhang, T. Zhan, G. Wu, J. Shen, and Z. Liu, (2012), Fusing the vegetative insecticidal protein Vip3Aa7 and the N terminus of Cry9Ca improves toxicity against Plutella xylostella larvae, Appl. Microbiol. Biotechnol, 96, p921-929.
[https://doi.org/10.1007/s00253-012-4213-y]
-
Eom, S., Y. Park, and Y. Kim, (2014), Sequential immunosuppressive activities of bacterial secondary metabolites from the entomopathogenic bacterium, Xenorhabdus nematophila, J. Microbiol, 52, p161-168.
[https://doi.org/10.1007/s12275-014-3251-9]
-
Gillespie, J. P., M. R. Kanost, and T. Trenczek, (1997), Biological mediators of insect immunity, Annu. Rev. Entomol, 42, p611-643.
[https://doi.org/10.1146/annurev.ento.42.1.611]
- Gho, H. K., S. G. Lee, B. P. Lee, K. M. Choi, and J. H. Kim, (1991), Simple mass-rearing of beet armyworm, Spodoptera exigua (Hbner) (Lepidoptera: Noctuidae), on an artificial diet, Kor. J. Appl. Entomol, 29, p180-183.
-
Grizanova, E. V., I. M. Dubovskiy, M. M. A. Whitten, and V. V. Glupov, (2014), Contributions of cellular and humoral immunity of Galleria mellonella larvae in defence against oral infection by Bacillus thuringiensis, J. Invertebr. Pathol, 119, p40-46.
[https://doi.org/10.1016/j.jip.2014.04.003]
-
Hwang, J., Y. Park, and Y. Kim, (2013), An entomopathogenic bacterium, Xenorhabdus nematophila, suppresses expression of antimicrobial peptides controlled by Toll and IMD pathways by blocking eicosanoid biosynthesis, Arch. Insect Biochem. Physiol, 83, p151-169.
[https://doi.org/10.1002/arch.21103]
-
Jung, S., and Y. Kim, (2006), Synergistic effect of entomopathogenic bacteria (Xenorhabdus sp. and Photorhabdus temperata ssp. temperata) on the pathogenicity of Bacillus thuringiensis ssp. aizawai against Spodoptera exigua (Lepidoptera: Noctuidae), Environ. Entomol, 35, p1584-1589.
[https://doi.org/10.1093/ee/35.6.1584]
-
Kaya, H. K., and R. Gaugler, (1993), Entomopathogenic nematodes, Annu. Rev. Entomol, 38, p181-206.
[https://doi.org/10.1146/annurev.en.38.010193.001145]
-
Kim, K., H. Kim, Y. Park, K. H. Kim, and Y. Kim, (2013), An integrated biological control using an endoparasitoid wasp (Cotesia plutellae) and a microbial insecticide (Bacillus thuringiensis) against the diamondback moth, Plutella xylostella, Kor. J. Appl. Entomol, 52, p35-43.
[https://doi.org/10.5656/KSAE.2013.01.1.080]
-
Kim, Y., D. Ji, S. Cho, and Y. Park, (2005), Two groups of entomopathogenic bacteria, Photorhabdus and Xenorhabdus, share an inhibitory action against phospholipase A2 to induce host immunodepression, J. Invertebr. Physiol, 89, p258-264.
[https://doi.org/10.1016/j.jip.2005.05.001]
-
Kirkpatrick, B. A., J. O. Washburn, and L. E. Volkman, (1998), AcMNPV pathogenesis and developmental resistance in fifth instar Heliothis virescens, J, Invertebr. Pathol, 72, p63-72.
[https://doi.org/10.1006/jipa.1997.4752]
-
Kwon, S., and Y. Kim, (2007), Immunosuppressive action of pyriproxyfen, a juvenile hormone analog, enhances pathogenicity of Bacillus thuringiensis subsp. kurstaki against diamondback moth, Plutella xylostella (Lepidoptera: Yponomeutidae), Biol. Control, 42, p72-76.
[https://doi.org/10.1016/j.biocontrol.2007.03.006]
-
Park, H. W., D. K. Bideshi, and B. A. Federici, (2005), Synthesis of additional endotoxins in Bacillus thuringiensis subsp. morrisoni PG-14 and Bacillus thuringiensis subsp. jegathesan significantly improves their mosquitocidal efficacy, J. Med. Entomol, 42, p337-341.
[https://doi.org/10.1093/jmedent/42.3.337]
- Park, J. W., and B. L. Lee, (2012), Insect immunology, In Insect molecular biology and biochemistry, L. I. Gilbert >Ed, Academic Press, New York, p480-512.
-
Park, Y., and Y. Kim, (2003), Xenorhabdus nematophilus inhibits p-bromophenacyl bromide (BPB)-sensitive PLA2 of Spodoptera exigua, Arch. Insect Biochem. Physiol, 54, p143-142.
[https://doi.org/10.1002/arch.10108]
-
Park, Y., Y. Kim, and D. Stanley, (2004a), The bacterium Xenorhabdus nematophila inhibits phospholipase A2 from insect, prokaryote, and vertebrate sources, Naturwissenschaften, 91, p371-373.
[https://doi.org/10.1007/s00114-004-0548-2]
-
Park, Y., Y. Kim, H. Tunaz, and D. W. Stanley, (2004b), An entomopathogenic bacterium, Xenorhabdus nematophila, inhibits hemocytic phospholipase A2 (PLA2) in tobacco hornworm, Manduca sexta, J. Invertebr. Pathol, 86, p65-71.
[https://doi.org/10.1016/j.jip.2004.05.002]
-
Rahman, M. M., H. L. S. Roberts, M. Sarjan, S. Asgari, and O. Schmidt, (2004), Induction and transmission of Bacillus thuringiensis tolerance in the flour moth Ephestia kuehniella, Proc. Natl. Acad. Sci. USA, 101, p2696-2699.
[https://doi.org/10.1073/pnas.0306669101]
- Roh, J. Y., J. Y. Choi, M. S. Li, B. R. Jin, and Y. H. Je, (2007), Bacillus thuringiensis as a specific, safe, and effective tool for insect pest control, J. Microbiol. Biotechnol, 17, p547-559.
- SAS Institute, Inc, (1989), SAS/STAT user's guide, Release 6.03, Ed, Cary, N.C.
-
Seo, S., S. Lee, Y. Hong, and Y. Kim, (2012), Phospholipase A2 inhibitors synthesized by two entomopathogenic bacteria, Xenorhabdus nematophila and Photorhabdus temperata subsp. temperata, Appl. Environ. Entomol, 78, p3816-3823.
[https://doi.org/10.1128/AEM.00301-12]
-
Shrestha, S., and Y. Kim, (2009), Biochemical characteristics of immune-associated phospholipase A2 and its inhibition by an entomopathogenic bacterium, Xenorhabdus nematophila, J. Microbiol, 47, p774-782.
[https://doi.org/10.1007/s12275-009-0145-3]
-
Singh, G., P. J. Rup, and O. Koul, (2007), Acute, sublethal and combination effects of azadirachtin and Bacillus thuringiensis toxins on Helicoverpa armigera (Lepidoptera: Noctuidae) larvae, Bull. Entomol. Res, 97, p351-357.
[https://doi.org/10.1017/s0007485307005019]
-
Stanley, D., and Y. Kim, (2014), Eicosanoid signaling in insects; from discovery to plant protection, Crit. Rev. Plant Sci, 33, p20-63.
[https://doi.org/10.1080/07352689.2014.847631]
-
Vojtech, E., M. Meissle, and G. M. Poppy, (2005), Effects of Bt maize on the herbivore Spodoptera littoralis (Lepidoptera: Noctuidae) and the parasitoid Cotesia marginiventris (Hymenoptera: Braconidae), Transgenic Res, 14, p133-144.
[https://doi.org/10.1007/s11248-005-2736-z]
-
Washburn, J. O., B. A. Kirkpatrick, and L. E. Volkman, (1995), Comparative pathogenesis of Autographa californica M nuclear polyhedrosis virus in larvae of Trichoplusia ni and Heliothis virescens, Virology, 209, p561-568.
[https://doi.org/10.1006/viro.1995.1288]
-
Washburn, J. O., J. F. Wong, and L. E. Volkman, (2001), Comparative pathogenesis of Helicoverpa zea S nucleopolyhedrovirus in noctuid larvae, J. Gen. Virol, 82, p1777-1784.
[https://doi.org/10.1099/0022-1317-82-7-1777]
-
Wirth, M. C., Y. Yang, W. E. Walton, B. A. Federici, and C. Berry, (2007), Mtx toxins synergize Bacillus spaericus and Cry11Aa against susceptible and insecticide-resistant Culex quinquefasciatus larvae, Appl. Environ. Microbiol, 73, p6066-6071.
[https://doi.org/10.1128/AEM.00654-07]
-
Zhang, X., M. Candas, N. B. Griko, R. Taussig, and L. A. Bulla Jr, (2006), A mechanism of cell death involving an adenylyl cyclase/PKA signaling pathway is induced by the Cry1Ab toxin of Bacillus thuringiensis, Proc. Natl. Acad. Sci. USA, 103, p9897-9902.
[https://doi.org/10.1073/pnas.0604017103]