
Screening Antifungal and Exceptional Colonization Strains from Nationwide Actinobacteria Library
Abstract
Since the Industrial Revolution, the population has grown exponentially and by 2050, the world's population is expected up to 9 billion. As the population increases, food supply is required to about 1.5 times the current amount, but the occurrence of diseases and environmental stress on crops due to climate change is increasing and crop production is decreasing. Chemical pesticides have contributed greatly to the increase in food production and security. However, due to issues of environmental, eco-friendly management techniques are demanded. There are many plants beneficial microorganisms that have been known to contribute to preventing plant disease occurrence as eco-friendly approaches in lab or greenhouse experimental scales. However, the candidate beneficial microbes failed to protect the plant against pathogens or abiotic stresses in commercial crop cultivated field conditions, due to a lack of colonization ability on plants. Therefore, this study aimed to secure outstanding colonization of microbial strains among Actinobacteria collection nationwide. Of the 2,114 isolates of Actinobacteria, two strains showed aggressive antifungal activity against a wide range of fungal pathogens and exceptional colonization ability in the plant rhizosphere. The strains have an excellent possibility to be biological control agents and members of the synthetic community for plant disease control.
Keywords:
Arabidopsis thaliana, Kitasatospora, Rhizosphere, StreptomycesIntroduction
Plants keep the Earth’s ecosystem alive by providing oxygen and habitats for wild animals, protecting from solar radiation, preventing soil erosion, and most importantly, providing food for humankind. Also, plants have many complicated interrelationships with other creatures, including microbial organisms. For example, more than 100,000 fungi, 500 bacteria, and 1,000 viruses are known to have interacted with the plants (Agrios, 2005). Among the microorganisms, certain species have evolved into pathogens that obtain nutrients from living plants. And the pathogens are known to destroy 20% to 30% of the world’s food production every year (Agrios, 2005). To secure food production, more than 2 million tons of chemical pesticides are used worldwide each year (Gill and Garg, 2014). The pesticides cause serious negative side effects such as decreased biodiversity, the biological concentration of the chemicals, poisoning of farmers, and most importantly, triggering the emergence of pesticide-resistance pathogens in a short period (Carson, 1962).
For this reason, research on plant disease control methods that replace pesticides or minimize the use of pesticides has been conducted for the past several decades. The best effective approach to replace chemical pesticides is to use microorganisms that have a beneficial effect on plants and inhibit pathogens. Plant growth-promoting bacteria (PGPB) demonstrated that PGPB can enhance plant growth and prevent disease occurrences (Cha et al., 2016; Jeon et al., 2021; Kim et al., 2019b; Thomashow et al., 2019). Among various shreds of evidence of PGPB, wheat take-all disease, caused by fungal pathogen Gaeumannomyces graminis var. tritici (Ggt), and take-all decline is the well-studied (Kwak and Weller, 2013). Pseudomonas brassicacearum Q8r1-96 is the key rhizosphere PGPB to suppress wheat take-all disease occurrence. 2,4-diacetylphlorglucinol, a natural antifungal agent produced by Q8r1-96, strongly inhibits Ggt growth and as a result, the take-all disease is suppressed (Yang et al., 2018; Weller, 2007). Streptomyces griseus S4-7 is well characterized as a responsible microbial agent against Fusarium wilt disease in strawberries (Cha et al., 2016). S4-7 produced several lantipeptide derivatives, among them conprimycin and grisin have bioactivity against fungal pathogens (Kim et al., 2019a; Kim and Kwak, 2021a).
Recently, the Genus of Streptomyces has been attracting attention for the suppression of plant diseases. Streptomyces spp. is ubiquitous soil bacteria and produces a variety of secondary metabolites. More than 80% of antibiotics, which used for clinical purposes and animal feeding, are originated from Streptomyces (Bentely et al., 2002; Harir et al., 2018). Plant-associated Streptomyces have been founded in the rhizosphere, phyllosphere, and even the endosphere of plant tissue (Kim et al., 2019a; Kim et al., 2021b). Now a day, various PGPB candidate microorganisms are being suggested through the plant microbiome community analyses, and these candidate agents are being attempted to control plant diseases (Cha et al., 2016; Kim et al., 2019b). Raaijmakers and Weller (1998) presented the milestone discovery that the colonization ability of the PGPB is the most critical factor to protect the plant against pathogen attack. Among PGPB candidate agents, many agents failed to protect plants against pathogen invasion in field conditions due to a lack of colonization ability.
The purposes of this study were to screen and select excellent root colonization strains from the Actinobacteria library to enhance the colonization of antipathogen stains in the synthetic community (SynCom) for plant disease management.
Materials and Methods
Soil sample collection and construction Actinobacteria library
Highland soil samples were collected from each peak of Mt. Seolark, Deokyu, Sobaek, Songni, Taebaek, and Wolak, which are included in the Baekdudaegan, a large mountain stem located in South Korea. Soil samples were collected separately from the soil in the area where people were passing (way) and the soil next to the road where they could not reach (wayside). At each sampling point, we collect approximately 15 gram of soil in a plastic bag using a disposable spoon and the GPS information was recorded. The coordinates and altitude of the peak where soil samples are collected can be found in the following Table S1. To isolate the cultivable microorganisms, soil samples diluted from 10-3-fold to 10-5-fold in sterile water were spread in quintuplicate onto tryptic soy agar (TSA: tryptic soy broth [Difco, Franklin Lakes, NJ, USA] 30 g, agar 20 g per 1 L) and incubated at 28oC for 5 days. Additionally, cycloheximide was added to suppress the growth of fungi (final concentration of 50 μg/mL). After 5 days, the number of colonies was counted for measuring the colony forming unit (CFU) value. The measured CFU value was visualized as bar graphs through ggplot2 (version 3.2.0) package (Ito and Murphy, 2013) in the R program (version 3.4.4).
To secure storage stocks for isolated Actinobacteria, only Actinobacteria was selected from among the colonies that were grown and re-cultivated using a needle on MS medium (soya flour, 20 g; mannitol, 20 g; agar, 20 g per 1 L) for five days. Afterward, uncontaminated Actinobacteria were secured, and each colony was inoculated in a 96-well plate with 100 μL of tryptic soy broth (TSB: tryptic soy broth 30 g per 1 L) for the bacterial stock library. After incubation for 4 days in a 28oC shaking incubator (170 rpm), 100 μL of 50% glycerol was added to each well. Then, the plates were stored at -80oC freezer after sealing with Platemax® pierceable aluminum sealing firm (Axygen, Union City, CA, USA). In the case of separated Actinobacteria isolates numbers were specified according to the name of the isolated peak, type of soil sample, and location of the storage stock of the 96-well plates (Fig. S1).
Confirm antifungal activity of isolated Actinobacteria strain
Antifungal activity of the Actinobacteria isolates from soil samples were confirmed by a three-step screening procedure. First, the storage stock of 96-well plates was replica-plated onto PDK medium (potato dextrose [Difco] 10 g, peptone 10 g, agar 20 g per 1 L) in Omni-trays (Sigma-Aldrich, St. Louis, MO, USA) and cultured at 28oC for 3 days. Then, 4-mm-diameter plugs of actively growing mycelia of Rhizoctonia solani were placed between the library colonies. After an additional three days of incubation, the first screening of the antifungal bacterial strain was selected, which suppressed the growth of R. solani. Second, colonies that inhibited fungal growth were inoculated into TSB broth and the concentration was adjusted to 1.0 of OD600. 10 μL of cultured bacteria were spotted onto PDK agar with regular intervals of each 8 strains per 1 petri dish. Three days later, a 4-mm-plug of the R. solani was placed at the center of the plate. Fungal suppression was evaluated 5 days later. Antifungal activity is defined based on the distance between the pathogen and the antagonist after 5 days. The third screening was conducted with 4 stains, which showed outstanding antifungal activity in the second screening. As with the second screening, the antifungal ability was identified through the inhibitory distance of the fungus (n=5) and denoted as - indicates no activity; + indicates a zone of inhibition on average under 7 mm (low inhibition), ++ indicates a zone of inhibition in the average of 7 to 9 mm (medium inhibition), +++ indicates a zone of inhibition in average over 10 mm (strong inhibition).
Based on the inhibitory ability against R. solani, the final selected strains were dual cultured with Botrytis cinerea, Colletotrichum gloeosporioides, Fusarium oxysporum, Phytophthora capsici to confirm the antagonism with other types of fungi. All pathogenic fungi were used from the lab collection. The antifungal ability verification was conducted in the same method as the third screening, with five repetitions per strain.
Adhesion activity with crystal violet, Congo red assay in vitro
Antifungal strains were cultured in 5 mL of minimal medium (2 g of (NH4)2SO4, 5 g of casamino acids, 0.6 g of MgSO4, 50 g of polyethylene glycol 6000, 1 mL of minor elements solution, 15 mM NaH2PO4, K2HPO4 buffer (pH 6.8), 0.5% glucose per 1 L) at 27oC for 10 days in 96-well plates. After 10 days, non-adherent bacterial cells were removed by washing with 5 mL of 1X PBS. 10 mL of each 1% crystal violet or 0.2% Congo red solution was added to each well and incubated at room temperature for 4 hours. All the solutions were completely removed using the 10 mL pipette and each tube was dried at room temperature overnight. Crystal violet and Congo red residues were solubilized with 2 mL of 95% HPLC ethanol. The turbidity was compared with control and OD570 was measured by spectroscopy. Statistically significant differences were indicated in ANOVA following the Duncan test at p = 0.05.
Root colonization assay in Arabidopsis thaliana Col-0
Based on the antifungal ability and outstanding attachment capability in vitro conditions, the selected strains were conjugated with pSET152, an apramycin-resistant plasmid, and Arabidopsis thaliana Col-0 was used to confirm root colonization capability. For seed disinfection, 1 mL of 1% NaOCl was added to a 1.5 mL-tube with the seed of A. thaliana. After vortexing, NaOCl was completely removed through centrifugation, and washed the seeds with sterilized water five times. After blocking the light, the seeds were kept at 4oC a refrigerator in 1 mL of sterilized water for 3 days. After the vernalization, the seed was planted on a paper disk soaked with sterilized water. Sprouted seeds were transplanted into a pot with autoclaved soil. The A. thaliana Col-0 was cultivated for 3 weeks, then 107CFU/mL of pSET152 (apramycin resistance gene contained plasmid) conjugated strain in 0.1% methylcellulose was drenched 3 times at 4-days intervals. From the final drenching, the root colonized the apramycin resistance strains in the rhizosphere of the A. thaliana was counted. MS media with apramycin (50 μg/mL of final concentration) was used to calculate CFU values of the pSET152 conjugated colonies. Additionally, the CFU values of target strains were measured up to 30 days. CFU values were calculated with 5 repetitions and visualized through ggplot2 in R program.
Identification of Actinomycetes by molecular characterization
The strains were cultured on MS agar media and when the colonies produced aerial hypha, were scratched out by a disinfected razor blade, and then transferred into a 1.5-mL tube. Genomic DNA was extracted using the CTAB method (Kutchma et al., 1998). To identify the strains, 6 genes were amplified and sequenced as follow: 16S rRNA (27f: 5'-AGAGTTTGATCCTGGCTCAG-3', 1492r: 5'- TACGGTTACCTTGTTACGACTT-3') atpD (atpDF: 5'- ACCAAGGGCAAGGTGTTCAA-3', atpDR: 5'-GCCGGGTAGATGCCCTTCTC-3'), gyrB (gyrBF-1: 5'-GAGGTCGTGCTGACCGTGCTGCA-3', gyrBF-4: 5'-CGCTCCTTGTCCTCGGCCTC-3'), recA (recAF: 5'-ACAGATTGAACGGCAATTCG-3’, recAR: 5'-ACCTTGTTCTTGACCACCTT-3'), ropB (ropBF1: 5'-TTCATGGACCAGAACAACC-3', ropBR1: 5'- CGTAGTTGTGACCCTCCC-3'), trpB (trpBF: 5'- GGCTCACACAAGATCAACAA-3', trpBR: 5'- TCGATGGCCGGGATGATGCC-3') (Guo et al., 2008). Preparation of amplicon reaction and polymerase chain reaction (PCR) conditions were followed by the previous reports (Kutchma et al., 1998; Guo et al., 2008). The PCR products were sequenced by Macrogen Inc. (Seoul, Republic of Korea) and the Basic Local Alignment Search Tool (BLAST: www.ncbi.nlm.nih.gov) was used to identify the bacterial strain. Phylogenetic trees were constructed from each amplicon sequence and multiple sequence alignments using the maximum likelihood algorithm in the MEGA 11 program.
Results and Discussion
Collection of highland soil samples and building Actinobacteria library
The highland soil samples were collected from Mt. Seolark, Mt. Deokyu, Mt. Sobeak, Mt. Taeback, Mt. Wolak, Mt. Songni (Table S1). Most of the sampling site altitude was higher than 1,000 meters, which indicated environmental conditions were different from those of agricultural land. The population density of culturable bacteria in most highland sampling sites was about 105 CFU/gram, regardless way or wayside (Fig. S2). From the culturable bacteria, we isolated a total of 2,144 Actinobacteria isolates based on colony morphological characters, and among them, 924 isolates were obtained from the way and 1,220 colonies were secured from the wayside (Table 1). The Actinobacteria isolates were cultured in 96-well plates as the collection library (Fig. S1). Actinobacteria are ubiquitous bacteria in soil, water, and even plants (Bentley et al., 2002). Most Actinobacteria collection and functional characterization have been conducted from various agricultural land sites. In this study, we generated the Actinobacteria collection library in highland mountains, which are environmentally severely harsh conditions, such as nutrients, temperature, and humidity. Therefore, there is a high possibility of selecting biologically superior strains from the highland Actinobacteria library (HAL).
Screening antifungal stains in the HAL
To screen antifungal strains in the HAL, we test a total of three evaluations against Rhizoctonia solani, a destructive necrotrophic fungal pathogen, which has a very broad host range. The first screening was conducted in a 96-well plate format, and 544 isolates were selected as candidate strains from a total of 2,144 isolates. During the second and third evaluations, a relatively small number of isolates were tested on a plate to enhance the clarity of the result. From the second test, 171 isolates were screened and from the third confrontation assay, 23 strains were selected as anti-R. solani (Fig. 1). Antifungal range test was performed with the selected 23 strains against major plant pathogenic fungi, such as Botrytis cinerea, Colletotrichum gloeosporioides, Fusarium oxysporum and Phytophthora capsici (Table 2 and Fig. 2). Interestingly, each strain showed different antifungal ranges. For example, SN1G12, SN2D2, SH1H6, SN1D6, and SN1G6 strains isolated from Mt. Songni (SN) had an antifungal effect against all tested fungal pathogens. However, SN1A10 and SN1F10 inhibited R. solani and P. capsica, and Strain JO75H showed antifungal activity only against R. solani. The result suggested that each strain has different biological properties in antifungal effect. Our finding indicated that to maximize the PGPB effect, we may need different combinations of PGPB strains in SynCom for application and academic proposes.
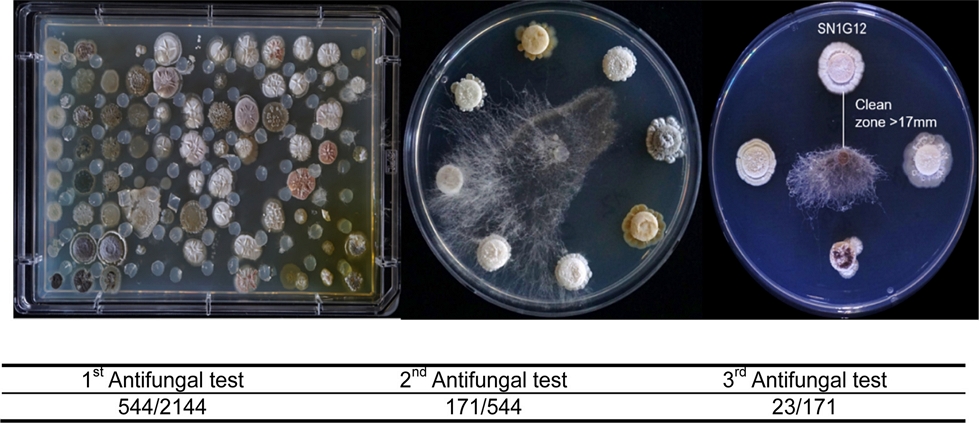
Library antifungal activity screening with Rhizoctonia solani. The first screening was performed on Omni-trays and the second and third screenings were evaluated on plates. After the fungus was placed on PDK agar media, the plates were incubated at 28oC for 3 days (The first screening) and 5 days (the second and third screening).

Antagonism tests with pathogenic fungi on PDK media at 28oC. In control, only fungi were inoculated. In Actinomyces spp. treatment, the isolates were inoculated first and three days after, the fungi were inoculated. Antifungal activity of the isolates was evaluated at 5 days. A: Rhizoctonia solani, B: Fusarium oxysporum, C: Botrytis cinerea, D: Colletotrichum gloeosprioids, E: Phytophthora capsica.
Attachment of the strains in vitro and colonization in planta
To select outstanding colonization stains, we performed two different assays. The first assay was in vitro biochemical tests with crystal violet and Congo red dyeing process. As a result of the adhesion tests using two dyeing systems, similar results were derived regardless of the type of dyeing chemicals (Fig. 3A and B). Among 23 antifungal strains, SN1G12 showed outstanding attachment ability (3.491 absorbance value [AV]) (Table 2 and Fig 3). Strains GU69F (2.927), JO610D (2.732), SN1A10 (2.434), HJ2F8 (2.375), and JO65A (2,228) had an adhesive AV of 2 or more, respectively.
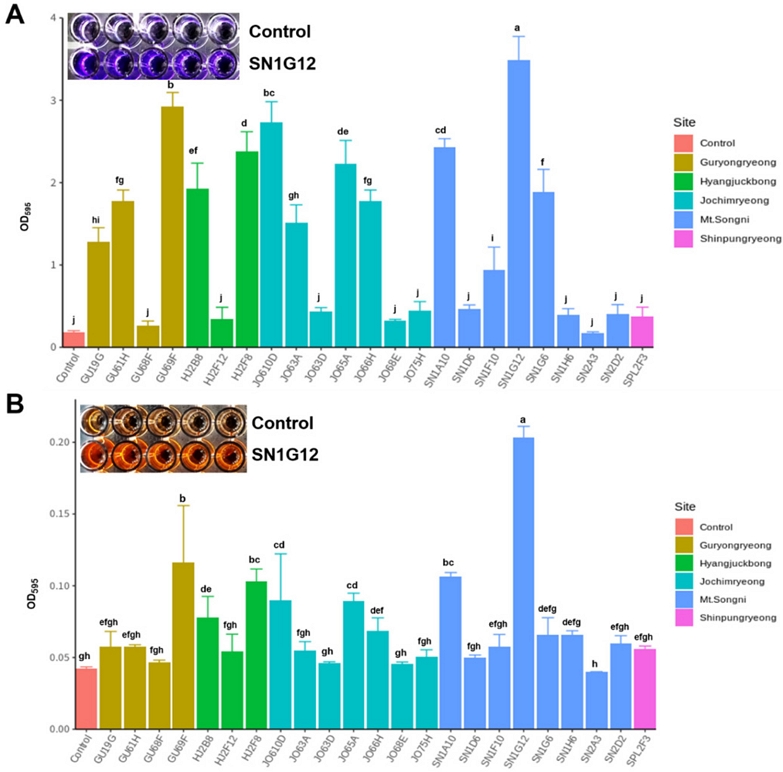
Adhesin assay of the strains. Strains were inoculated initial concentration at Optical density (OD) value 0.2 in 1/5 PDK broth for 5 days. After 5days, each dye was added to preempted 96 well plates where the bacteria were previously inoculated. Three hours later the dyes were cleaned using PBS solution, and 100% ethanol was added to measure the OD value. OD value was measured at wavelength 595. A: 1% Crystal violet, B: 0.2% Congo red. Each treatment contains 5 replicates. Statistical differences indicated ANOVA (Duncan test at p = 0.05).
Among the 6 strains, which showed an AV of 2 or higher, two strains SN1G12 and HJ2F8 had broad antifungal activity against various pathogens. Therefore, in plant colonization evaluation was used the SN1G12 and HJ2F8 strains and A. thaliana Col-0. Genetically antibiotic resistance marked SN1G12 and HJ2F8 (1 × 107 CFU/mL) were drenched on the three-week-old Col-0 plants 3 times. Both SN1G12 and HJ2F8 were confirmed to colonize well for up to 30 days in the rhizosphere of A. thaliana Col-0 (Fig. 4).

Colonization of the strain on Arabidopsis thaliana Col-0 root. Bacteria cell (107 CFU/mL) in 0.1% methylcellulose were drenched 3 times at interval of 4 days. A. thaliana Col-0 were planted at 28oC growth chamber. The bacteria density was evaluated on apramycin (50 μg/mL) contained MS media. CFU values were calculated with 5 repetitions and visualized through ggplot2 in R program.
These findings suggested that in vitro attachment assay approach is quite a reliable method to screen excellent adhesion microorganisms from the random collection. Because the SN1G12 and HJ2F8 presented aggressive adhesion effects on the plastic surface, and also exceptional colonization ability in the plant rhizosphere, even the surface chemical and structure conditions are quite different. PGPB can protect and enhance plants against pathogen challenges through various mechanisms, such as nutrient competition, antibiotic production, iron scavenging in soil, parasitism, induced systemic resistance, and production of plant hormones (Kushwaha et al., 2020; Weller, 2007). Regardless mechanisms of PGPB, maintaining population density in the rhizosphere is the most critical factor of PGPB (Raaijmakers and Weller, 1998; Yang et al., 2018). Especially, the SN1G12 and HJ2F8 strains maintained a very high population density in the rhizosphere of A. thaliana. Therefore, the SN1G12 and HJ2F8 were confirmed to have very reliable potential to be developed as biological control agents in plant disease management.
Identification stains
A total of 6 housekeeping genes were employed to identify the strains SN1G12 and HJ2F8 (Fig. S3). The genes were 16S rRNA, atpD, gyrB, recA, ropB, and trpB (Guo et al., 2008) SN1G12 was classified as a species of Streptomyces, and the HJ2F8 strain belonged to the Genus of Kitasatospora. Based on the single gene sequences alignment and even the multilocus phylogeny result also did not present the perfect match with the previously known strains (Fig. 5). This finding suggested that SN1G12 and HJ2F8 strains may be a new species of Actinobacteria and required a whole genome sequence approach to obtain clear identification of the strains.
Acknowledgments
This work was supported by the National Research Foundation of Korea (NRF) grant funded by the Korea government (MIST) [2020R1A2C2004177] and the Rural Development Administration (PJ015871).
Conflicts of Interest
The authors declare that there is no conflict of interest
References
- Agrios G, 2005. Plant Pathology. 5th Edition, Elsevier Academic Press, New York, USA.
- Bentley SD, Chater KF, Cerdeño-Tárraga A-M, Challis GL, Thomson NR, et al., 2002. Complete genome sequence of the model actinomycete Streptomyces coelicolor A3(2). Nature 417:141-147.
- Carson R, 1962. Silent spring. Houghton Mifflin, Boston, USA
-
Cha J, Han S, Hong H, Cho H, Kim D, et al., 2016. Microbial and biochemical basis of a Fusarium wilt-suppressive soil. ISME J. 10:119-129.
[https://doi.org/10.1038/ismej.2015.95]
- Gill H, Garg H, 2014. Pesticide: Environmental impacts and management strategies. In Pesticides: Toxic aspects, eds. By Larramendy M. and Soloneski, S. pp.187-229, InTech, Rijecka, Croatia.
-
Guo Y, Zheng W, Rong X, Huang Y, 2008. A multilocus phylogeny of the Streptomyces griseus 16S rRNA gene clade: use of multilocus sequence analysis for Streptomycete systematics. Int. J. Syst. Evol. Microbiol. 58:149-159.
[https://doi.org/10.1099/ijs.0.65224-0]
-
Harir M, Bendif H, Bellahcene M, Fortas Z, Pogni R, 2018. Streptomyces secondary metabolites. In: Basic biology and applications of actinobacteria, ed. by E. Shymaa, pp. 99-122. IntechOpen, London, UK.
[https://doi.org/10.5772/intechopen.79890]
-
Ito K, Murphy D, 2013. Application of ggplot2 to pharmacometrics graphics. CPT Pharmacometrics Syst. Pharmacol. 2(10):1-16.
[https://doi.org/10.1038/psp.2013.56]
-
Jeon C, Kim D, Bae E, Kwak Y, 2021. Changes in bacterial community structure and enriched functional bacteria associated with turfgrass monoculture. Front. Bioeng. Biotechnol. 8:530067.
[https://doi.org/10.3389/fbioe.2020.530067]
-
Kim D, Jeon C, Shin J, Weller DM, Thomashow L, et al., 2019a. Function and distribution of a lantipeptide in strawberry Fusarium wilt disease-suppressive soil. Mol. Plant-Microbe Interact. 32(3):306-312.
[https://doi.org/10.1094/MPMI-05-18-0129-R]
-
Kim D, Cho G, Jeon C, Weller DM, Thomashow LS, et al., 2019b. A mutualistic interaction between Streptomyces bacteria, strawberry plants and pollinating bees. Nat. Commun. 10:4802.
[https://doi.org/10.1038/s41467-019-12785-3]
-
Kim D, Kwak Y, 2021a. A genome-wide analysis of antibiotic producing genes in Streptomyces globisporus SP6C4. Plant Pathol. J. 37(4):389-395.
[https://doi.org/10.5423/PPJ.NT.03.2021.0047]
-
Kim D, Jeon C, Cho G, Thomashow LS, Weller DM, et al., 2021b. Glutamic acid reshapes the plant microbiota to protect plants against pathogens. Microbiome 9(244).
[https://doi.org/10.1186/s40168-021-01186-8]
-
Kim D, Kim S, Lee SI, Kwak Y, 2022. Microbiota communities of heathy and bacterial pustules diseased soybean. Plant Pathol. J. 38:372-382.
[https://doi.org/10.5423/PPJ.OA.05.2022.0067]
-
Kutchma AJ, Roberts MA, Knaebel DB, Crawford DL, 1998. Small-scale isolation of genomic DNA from Streptomyces mycelia or spores. BioTechniques 24:452-457.
[https://doi.org/10.2144/98243st05]
-
Kushwaha RK, Rodrigues V, Kumar V, Patel H, Raina M, et al., 2020. Soil microbes-medicinal plants interactions: ecological diversity and future prospect. In: Plant Microbe Symbiosis. eds. by Varma, A., Tripathi, S., Prasad, R. Springer, Switzerkland.
[https://doi.org/10.1007/978-3-030-36248-5_14]
-
Kwak Y, Weller DM, 2013. Take-all of wheat and natural disease suppression: A review. Plant Pathol. J. 29(2):125-135.
[https://doi.org/10.5423/PPJ.SI.07.2012.0112]
- Raji AI, Möller C, Litthauer D, van Heerden E, Piater LA, 2008. Bacterial diversity of biofilm samples from deep mines in South Africa. Biokemistri 20:53-62
-
Raaijmakers JM, Weller DM, 1998. Natural plant protection by 2,4-diacetylphloroglucinol-producing Pseudomonas spp. in take-all decline soils. Mol. Plant-Microbe Interact. 11(2):144-152.
[https://doi.org/10.1094/MPMI.1998.11.2.144]
-
Thomashow LS, LeTourneau MK, Kwak Y, Weller DM, 2019. The soil-borne legacy in the age of the holobiont. Microb. Biotechnol. 12(1):51-54.
[https://doi.org/10.1111/1751-7915.13325]
-
Weller DM, 2007. Psudomonas biocontrol agents of soilborne pathogens: Looking back over 30 years. Phytopathology 97(2):250-256.
[https://doi.org/10.1094/PHYTO-97-2-0250]
-
Yang M, Mavrodi DV, Thomashow LS, Weller DM, 2018. Differential response of wheat cultivars to Pseudomonas brassicacearum and take-all decline soil. Phytopathology 108(12):1363-1372.
[https://doi.org/10.1094/PHYTO-01-18-0024-R]
Appendix
Supplementary information
Youn-Sig Kwak, Heeil Do, Da-Ran Kim
The supplementary information contains 1 table and 3 figures.
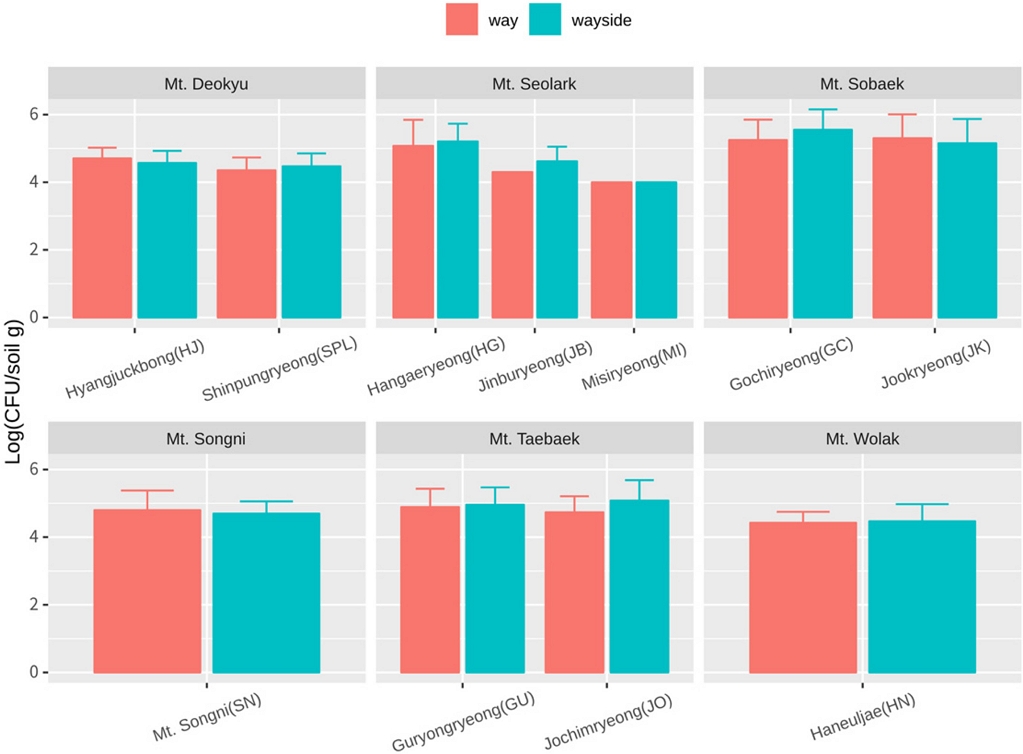
Total microbial population densities. Soil sample were separated by way and wayside. Bacteria were isolated from 8 different points in Hyangjuckbong (HJ), 7 different points in Shinpungryeong (SPL), Guryongryeong (GU), Jochimryeong (JO), 6 different points in Gochiryeong (GC), Jookryeong (JK), 4 different points in Mt. Songni (SN), Haneuljae (HN), 2 different points in Misiryeong (MI), 1 poin t in Hangaeryeong (HG), Jinburyeong (JB). Mt. Seolark (HG, JB, MI) contain 9 replicates of each point. Others contain 3 replicates of each point.
Youn-Sig Kwak, Research Institute of Life Science, Gyeongsang National University, Professor, http://orcid.org/0000-0003-2139-1808
Heeil Do, Division of Applied Life Science (BK21Plus), Gyeongsang National University, graduate student
Da-Ran Kim, Research Institute of Life Science, Gyeongsang National University, Research Professor, http://orcid.org/0000-0003-3460-901X